By:
Fabio Bergamin
Researchers at ETH Zurich have refined the famous
CRISPR-Cas method. Now, for the very first time, it is possible to
modify dozens, if not hundreds, of genes in a cell simultaneously.
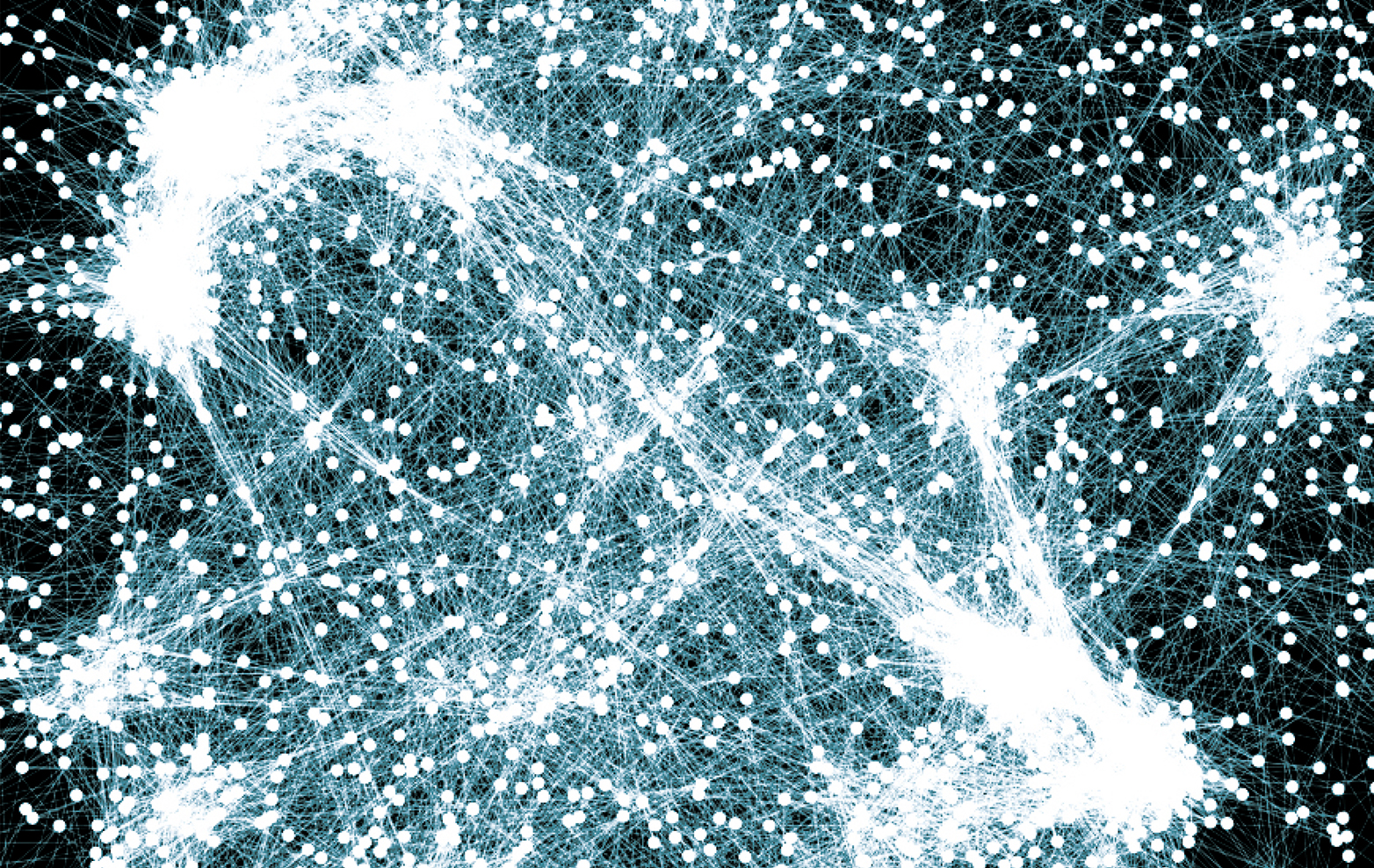
Genes and proteins
in cells interact in many different ways. Each dot represents a gene;
the lines are their interactions. For the first time, the new method
uses biotechnology to influence entire gene networks in one single step.
(Visualizations: ETH Zurich / Carlo Cosimo Campa)
Everyone’s talking about CRISPR-Cas. This
biotechnological method offers a relatively quick and easy way to
manipulate single genes in cells, meaning they can be precisely deleted,
replaced or modified. Furthermore, in recent years, researchers have
also been using technologies based on CRISPR-Cas to systematically
increase or decrease the activity of individual genes. The corresponding
methods have become the worldwide standard within a very short time,
both in basic biological research and in applied fields such as plant
breeding.
To date, for the most part, researchers could modify only one
gene at a time using the method. On occasion, they managed two or three
in one go; in one particular case, they were able to edit seven genes
simultaneously. Now, Professor Randall Platt and his team at the
Department of Biosystems Science and Engineering at ETH Zurich in Basel
have developed a process that – as they demonstrated in experiments –
can modify 25 target sites within genes in a cell at once. As if that
were not enough, this number can be increased still further, to dozens
or even hundreds of genes, as Platt points out. At any rate, the method
offers enormous potential for biomedical research and biotechnology.
“Thanks to this new tool, we and other scientists can now achieve what
we could only dream of doing in the past.”
Targeted, large-scale cell reprogramming
Genes and proteins in cells interact in many different ways.
The resulting networks comprising dozens of genes ensure an organism’s
cellular diversity. For example, they are responsible for
differentiating progenitor cells to neuronal cells and immune cells.
“Our method enables us, for the first time, to systematically modify
entire gene networks in a single step,” Platt says.
Moreover, it paves the way for complex, large-scale cell
programming. It can be used to increase the activity of certain genes,
while reducing that of others. The timing of this change in activity can
also be precisely controlled.
This is of interest for basic research, for example in
investigating why various types of cells behave differently or for the
study of complex genetic disorders. It will also prove useful for cell
replacement therapy, which involves replacing damaged with healthy
cells. In this case, researchers can use the method to convert stem
cells into differentiated cells, such as neuronal cells or
insulin-producing beta cells, or vice versa, to produce stem cells from
differentiated skin cells.
The dual function of the Cas enzyme
The CRISPR-Cas method requires an enzyme known as a Cas and a
small RNA molecule. Its sequence of nucleobases serves as an “address
label”, directing the enzyme with utmost precision to its designated
site of action on the chromosomes. ETH scientists have created a
plasmid, or a circular DNA molecule, that stores the blueprint of the
Cas enzyme and numerous RNA address molecules, arranged in sequences: in
other words, a longer address list. In their experiments, the
researchers inserted this plasmid into human cells, thereby
demonstrating that several genes can be modified and regulated
simultaneously.
For the new technique, the scientists did not use the Cas9
enzyme that has featured in most CRISPR-Cas methods to date, but the
related Cas12a enzyme. Not only can it edit genes, it can also cut the
long “RNA address list” into individual “address labels” at the same
time. Furthermore, Cas12a can handle shorter RNA address molecules than
Cas9. “The shorter these addressing sequences are, the more of them we
can fit onto a plasmid,” Platt says.
Reference
Campa CC, Weisbach NR, Santinha AJ, Incarnato D, Platt RJ:
Multiplexed genome engineering by Cas12a and CRISPR arrays encoded on
single transcripts. Nature Methods, 12 August 2019, doi: 10.1038/s41592-019-0508-6